
Since the Big Bang, the early Universe had hydrogen, helium, and a scant amount of lithium. Later, some heavier elements, including iron, were forged in stars. But one of the biggest mysteries in astrophysics is: How did the first elements heavier than iron, such as gold, get created and distributed throughout the Universe? In new research, astronomers from Columbia University and elsewhere calculated that a single flare from a magnetar can produce the mass equivalent of 27 moons’ worth of these elements in one go.
An artist’s impression of a magnetar. Image credit: NASA’s Goddard Space Flight Center / S. Wiessinger.
For decades, astronomers only had theories about where some of the heaviest elements in nature, like gold, uranium and platinum, come from.
But by taking a fresh look at old archival data, researchers now estimate that up to 10% of these heavy elements in the Milky Way are derived from the ejections of highly magnetized neutron stars, called magnetars.
“Until recently, astronomers had unwittingly overlooked the role that magnetars, essentially dead remnants of supernovae, might play in early galaxy formation,” said Ohio State University’s Professor Todd Thompson.
“Neutron stars are very exotic, very dense objects that are famous for having really big, very strong magnetic fields. They’re close to being black holes, but are not.”
While the origins of heavy elements had long been a quiet mystery, scientists knew that they could only form in special conditions through a method called the r-process (or rapid-neutron capture process), a set of unique and complex nuclear reactions.
They saw this process in action when they detected the collision of two super-dense neutron stars in 2017.
This event, captured using NASA telescopes, the Laser Interferometer Gravitational wave Observatory (LIGO) and other instruments, provided the first direct evidence that heavy metals were being created by celestial forces.
But further evidence showed that other mechanisms might be needed to account for all these elements, as neutron star collisions might not produce heavy elements fast enough in the early Universe.
Building on these clues helped Professor Thompson and his colleagues recognize that powerful magnetar flares could indeed serve as potential ejectors of heavy elements, a finding confirmed by 20-year-old observations of the SGR 1806-20 magnetar flare.
By analyzing this flare event, the researchers determined that the radioactive decay of the newly created elements matched up with their theoretical predictions about the timing and types of energies released by a magnetar flare after it ejected heavy r-process elements.
“This is really just the second time we’ve ever directly seen proof of where these elements form, the first being neutron star mergers,” said Columbia University’s Professor Brian Metzger.
“It’s a substantial leap in our understanding of heavy elements production.”
“It’s pretty incredible to think that some of the heavy elements all around us, like the precious metals in our phones and computers, are produced in these crazy extreme environments,” said Anirudh Patel, a doctoral candidate at Columbia University.
The researchers also theorized that magnetar flares produce heavy cosmic rays, extremely high-velocity particles whose physical origin remains unknown.
“I love new ideas about how systems work, how new discoveries work, how the Universe works,” Professor Thompson said.
“That’s why results like this are really exciting.”
The team’s paper was published in the Astrophysical Journal Letters.
_____
Anirudh Patel et al. 2025. Direct Evidence for r-process Nucleosynthesis in Delayed MeV Emission from the SGR 1806-20 Magnetar Giant Flare. ApJL 984, L29; doi: 10.3847/2041-8213/adc9b0
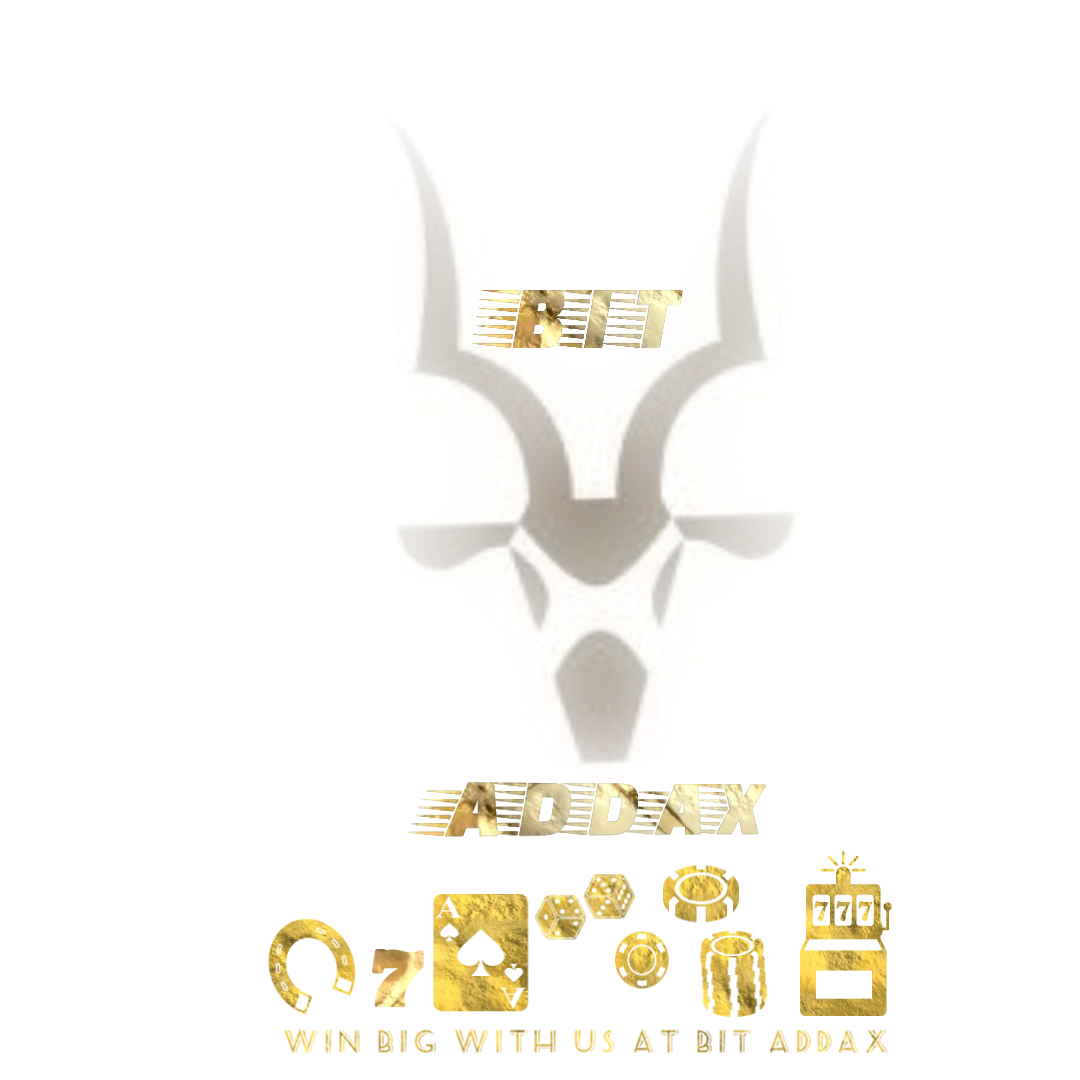